Chromosomes Show Off their Shapes; Super SQUID; Not So Dumb
Chromosomes Show Off their Shapes
Chromosomes — the 46 tightly wrapped packages of genetic material in our cells — are iconically depicted as X-shaped formations. However, those neat X’s only appear when a cell is about to divide and the entire contents of its genome duplicated. Until now, researchers have not been able to get a good picture of the way that our DNA — some two meters of strands, all told — is neatly bundled into the nucleus while enabling day-to-day (non-dividing) gene activity. A combination of new techniques for sequencing DNA in individual chromosomes and analyzing data from thousands of measurements has given us a new picture of the three-dimensional (3D) structures of chromosomes. This method, reported recently in Nature, is the result of an international collaboration that promises to help researchers understand the basic processes by which gene expression is regulated and genome stability is maintained.
Prof. Amos Tanay, who has dual appointments in the Weizmann Institute of Science’s Department of Computer Science and Applied Mathematics and Department of Biological Regulation, develops advanced computer algorithms for analyzing genomic datasets, which can run to billions of bits of information. He and his team, including doctoral students Yaniv Lubling and Eitan Yaffe, joined forces with Dr. Peter Fraser of the Babraham Institute, UK, in an attempt to resolve chromosomal architectures at an unprecedentedly high resolution. Rather than the traditional microscopy techniques, they harnessed the power of modern high-throughput DNA sequencing. Dr. Fraser and his team developed a sophisticated sequencing method for taking thousands of measurements of the contacts between genes inside single cells. While these techniques vastly improve upon approaches that average the conformations of millions of chromosomes, the data generated from just the few trillionths of a gram of DNA present within a single cell can only be interpreted by advanced statistical methods. That’s where Prof. Tanay and his team came in; they performed the complex computer analysis that turned millions of DNA sequences into reliable maps describing contacts between genes along individual chromosomes. With these maps, the team — in collaboration with Dr. Ernest Laue of Cambridge University — was able to produce 3D models of individual chromosome structures.
Interestingly, the new high-resolution depictions of chromosomal architecture indicate that the structure of the same DNA molecule can vary markedly between different cells. At the same time, the results point to some basic principles that underlie the genes’ organization. Their arrangement appears to be modular and based on the functions of the thousands of genes embedded within each chromosome. The data suggest that chromosomes expose the more active genes at their boundaries, possibly allowing these genes better access to the cellular machinery that regulates them.
Besides giving us a unique, surprising view of the structure of the chromosomes in our cells, the researchers believe that their method will give genetics research a powerful new tool. For example, it may help uncover variations in genetic activity between different types of cells, or promote understanding of the mechanisms determining gene activity or quiescence in various normal or disease conditions. The rapidly increasing power of massive DNA sequencing promises to make studies such as this even more beneficial in the near future.
Prof. Amos Tanay’s research is supported by the Helen and Martin Kimmel Award for Innovative Investigation; Pascal and Ilana Mantoux, Israel/France; the Wolfson Family Charitable Trust; the Rachel and Shaul Peles Fund for Hormone Research; and the estate of Evelyn Wellner.
Super SQUID
Weizmann Institute scientists have taken a quantum leap toward understanding the phenomenon known as superconductivity: They have created the world’s smallest SQUID — a device used to measure magnetic fields — which has broken the world record for sensitivity and resolution.
Superconductivity is a quantum phenomenon that only occurs when certain materials are cooled to extremely low temperatures. At that point, they lose all resistance to the flow of electricity and expel the magnetic fields within them. Although used in everything from magnetic resonance imaging (MRI) scanners to particle accelerators, scientists still do not fully understand the physics that underlies the behavior of superconductors. Among other things, superconducting materials are found in the very SQUIDs used to measure superconducting properties: SQUID stands for Superconducting QUantum Interference Device.
Nano-SQUIDs are placed on probes to scan and measure the magnetic field at different points on a sample, forming an image of the entire surface — a bit like creating a heat map of a hand by measuring its temperature at individual points on the fingers and palms.
Even very sensitive SQUIDS present geometric challenges when it comes to scanning materials: They need to be as small as possible to attain the highest image resolution, and they need to get as close as possible to the sample to image the tiniest magnetic features. Postdoctoral fellows Drs. Yonathan Anahory and Denis Vasyukov, PhD student Lior Embon, and their colleagues in the lab of Prof. Eli Zeldov of the Department of Condensed Matter Physics, have risen to the challenge — as reported in Nature Nanotechnology — thanks to a unique setup: They took a hollow quartz tube, pulled it into a very sharp point, then succeeded in fabricating a SQUID encircling the tip and measuring a mere 46 nanometers (nm) in diameter — the smallest SQUID to date. They then constructed a scanning microscope around the tip — an achievement that enabled them to obtain magnetic images at distances as small as a few nanometers from the sample. While current SQUID manufacturing methods limit their size and their ability to get very close to a surface, “We have the opposite problem: We have to prevent the probe from ‘crashing’ into the sample,” says Embon. “While there are SQUIDs with higher sensitivities to uniform magnetic fields, the combination of high sensitivity, proximity of the probe to the sample, and its minute dimensions make the overall accuracy of the device record-breaking.” This “nano-SQUID-on-tip” might, in the future, be able to measure the magnetic field from the spin of a single electron — the Holy Grail of magnetic imaging.
According to Prof. Zeldov, who is already using the new device to investigate superconductive phenomena in his lab, this invention will hopefully not only lead to a better understanding of superconductivity and vortex flow for the effective application of superconductor technology, but will aid in gaining insights into novel physical phenomena. As a surprising, added bonus, the new SQUID appears to be able to measure many materials other than superconductors.
Prof. Eli Zeldov is the incumbent of the David and Inez Myers Professorial Chair.
Not So Dumb
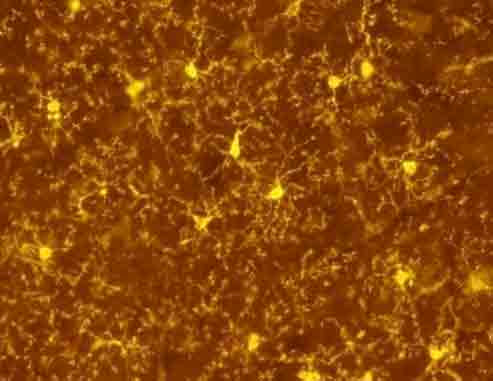
Microglia cells obtained using a mouse model developed by Prof. Steffen Jung’s team. Courtesy Weizmann Institute of Science.
Mysterious brain cells called microglia are starting to reveal their secrets, thanks to research conducted at the Weizmann Institute of Science.
Until recently, most of the glory in brain research went to neurons. For more than a century, these electrically excitable cells were believed to perform the entirety of the information processing that makes the brain such an amazing machine. In contrast, cells called glia — which together account for about half of the brain’s volume — were thought to be mere fillers that provided the neurons with support and protection but performed no vital function of their own. In fact, they had been named glia, the Greek for “glue,” precisely because they were considered so unsophisticated.
But in the past few years, the glia cells — particularly the tiny microglia that make up about one-tenth of the brain cells — have been shown to play critical roles both in the healthy and the diseased brain.
The octopi-like microglia are immune cells that conduct ongoing surveillance, swallowing cellular debris or, in the case of infection, microbes, to protect the brain from injury or disease. But these remarkable cells are more than just cleaners: In the past few years, they have been found to be involved in shaping neuronal networks by pruning excessive synapses — the contact points that allow neurons to transmit signals — during embryonic development. They are probably also involved in reshaping the synapses as learning and memory occurs in the adult brain. Defects in microglia are believed to contribute to various neurological diseases, among them Alzheimer’s disease and amyotrophic lateral sclerosis (ALS, or Lou Gehrig’s disease). By clarifying how exactly the microglia operate on the molecular level, scientists might be able to develop new therapies for these disorders.
More than a decade ago, the Weizmann Institute’s Prof. Steffen Jung developed a transgenic mouse model that, for the first time, enabled scientists to visualize the highly active microglia in the live brain. Now Prof. Jung has made a crucial next step: His laboratory developed a system for investigating the functions of microglia.
The scientists have equipped mice with a genetic switch: an enzyme that can rearrange previously marked portions of the DNA. The switch is activated by a drug, and when the mouse receives the drug, the enzyme performs a genetic manipulation — for example, to disable a particular gene. The switch is designed so that over the long term, it targets only the microglia, but not other cells in the brain or in the rest of the organism. In this manner, researchers can clarify not only the function of the microglia, but the roles of different genes in their mechanism of action.
As reported in Nature Neuroscience, Weizmann scientists, in collaboration with the team of Prof. Marco Prinz at the University of Freiburg, Germany, recently used this system to examine the role of an inflammatory gene expressed by the microglia. They found that the microglia contribute to an animal disease equivalent of multiple sclerosis. Prof. Jung’s team included Diana Varol, Yochai Wolf, and Dr. Simon Yona, all of Weizmann’s Department of Immunology.
The system developed at the Weizmann Institute, currently applied in numerous other studies by researchers at Weizmann and elsewhere, promises to shed new light on the role of the microglia in the healthy brain as well as the diseased brain with Alzheimer’s, ALS, and more.
Prof. Steffen Jung’s research is supported by the Leir Charitable Foundations; the Leona M. and Harry B. Helmsley Charitable Trust; the Adelis Foundation; Lord David Alliance, CBE; the Wolfson Family Charitable Trust; the estate of Olga Klein Astrachan; and the European Research Council.